
Getting to the roots of glioblastoma
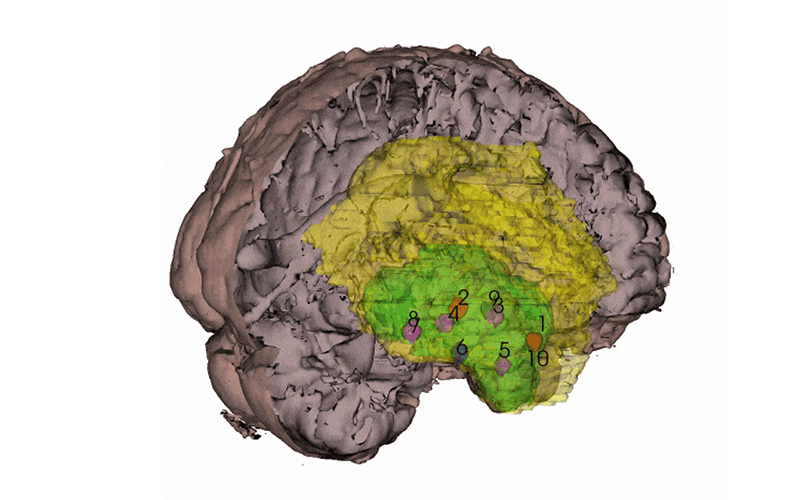
3D representations of individual patients’ brain tumors reveal origins, new potential vulnerabilities
Glioblastomas often grow back after initial treatment because the currently available therapies only manage to attack a portion of cells present within the tumor. Identifying more targeted treatment strategies requires a more complete understanding of the whole tumor landscape.
Researchers and clinicians at UC San Francisco — in collaboration with Feng Yue at Northwestern University, Marco Gallo at the University of Calgary, and Paul Boutros at UCLA — have charted the unique patterns of genetic and epigenetic alterations present throughout the tumor. Their study, published in Cell on January 18, offers a comprehensive map showing how these malignant tumors form and continue growing.
“If we could develop a therapy based on the knowledge that the target is everywhere on the tumor, then we get around that major concern about intratumoral heterogeneity,” said Joseph Costello, PhD, a neurosurgery professor at UCSF and one of the study’s co-senior authors.
By coordinating with neurosurgeons at the UCSF Brain Tumor Center and scientists at the Neurosurgery Biorepository, Costello and his colleagues obtained at least 10 samples each from 10 patients with newly diagnosed glioblastoma. They also asked the surgeons for samples widely distributed across the tumor — from the core all the way out to the periphery. With the neuroimaging expertise of radiology professor Janine Lupo, PhD, the researchers then generated 3D models of each individual patient’s tumor using the coordinates from the surgical neuronavigation software and pre-operative MRI scans.
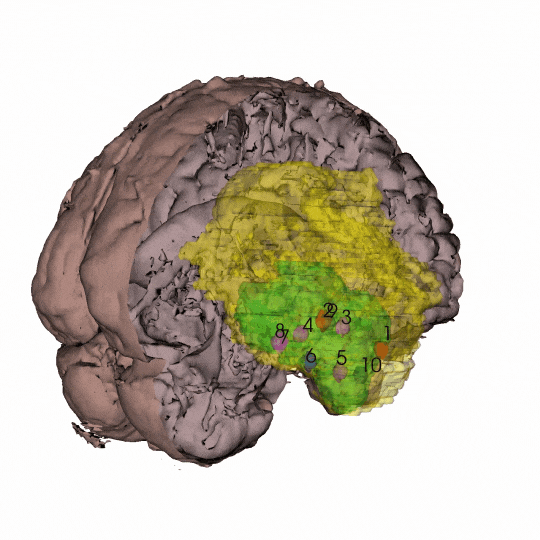
“Our spatial sampling approach was very unique because most people only have one sample, and then they try to extrapolate from that to the whole tumor,” said Radhika Mathur, PhD, a postdoctoral scholar in the Costello lab and the study’s first author.
The scientists used these 3D representations of each patient's tumor to situate their data about genetic and epigenetic alterations present in each sample within the context of the whole tumor. By controlling for how many cancer cells were in each sample, Mathur says their map also more accurately distinguishes between the tumor and its microenvironment.
This approach allowed the scientists to tease out features common among all the samples from the patients’ tumors.
Mathur says their data shows that genes important in neurodevelopment are active in the tumor cells. These results builds on the large body research showing the cells from which glioblastomas originate, specifically outer radial glial differentiation into oligodendrocyte precursor cells.
“Understanding cell-of-origin is so critical to figuring out which cells to use to develop a model of the disease,” Costello said. “If your model is more representative of the cells that give rise to tumor, then, presumably testing a therapy on them could be more predictive of patient response.”
To better understand what causes the cell-of-origin to undergo transformation into a cancer cell, he and his team applied a specialized genomics technique called Hi-C to examine the structure of the DNA in the cells from each sample.
“It is pretty striking because large portions of the chromosome are shattered and misassembled,” Mathur said.
“These types of DNA variants have been described in glioblastoma, but what we see is that it is in every sample of the tumor,” Costello added. “We have basically put chromosome shattering in the very beginning of how the tumor evolves.”
The structural changes in the DNA also result in fusion genes that can generate small proteins or peptides, which he says present new potential therapeutic opportunities. For example, cancer vaccines against these proteins could be used stimulate the immune system to attack to the tumor.
“We are really excited about the possibility of personalized immunotherapy,” Costello said, adding that his lab is collaborating on projects led by the laboratory of Hideho Okada, MD, PhD, to jump start the process.
Additionally, the researchers found that at the edges of the tumors, the cells expressed genes facilitating the communication with the surrounding healthy neurons.
These findings independently confirm previously published studies in the emerging field of cancer neuroscience. But their analysis of the gene expression patterns in the various tumor samples identifies many more genes that may be critical to the process.
The researchers have created a free online resource for other scientists to identify other possible tumor-wide drug targets and see where specific genes, programs, and cell types are active across glioblastomas.
This dataset is also just the beginning for Costello and his colleagues as they continue investigating the cellular events that cause malignant brain tumors to form. The next phase of the project will incorporate tumor samples from patients with lower-grade gliomas as well as patients with recurrent tumors.
Reference: Mathur et al, Glioblastoma Evolution and heterogeneity from a 3D whole-tumor perspective. Cell (2024). https://doi.org/10.1016/j.cell.2023.12.013